Research: Atomization
Turbulent Liquid Atomization
In most energy conversion systems, fuel is injected in liquid form. Atomization of the liquid fuel, or the process by which a coherent liquid flow disintegrates into droplets, represents one of the key challenges that remain to be tackled to make predictive simulations possible. Because atomization governs the size of the fuel droplets, and therefore their subsequent evaporation rate, it will have far-reaching repercussions on many aspects of the combustion process, for example pollutant formation. However, the inherent multi-physics and multi-scale nature of this process limits both experimental and numerical investigations.
The animation shows the turbulent atomization of a liquid jet at Re=3000 and We=2000. For this simulation, a spectrally refined level set approach is used to track the interface geometry, and ghost fluid is used to model surface tension forces and to account for the discontinuous material properties between the phases.
This simulation was conducted on 70 million grid points using 512 processors for two days. Such numerical studies can help better understand the fundamental physical processes behind atomization, ultimately allowing the development of accurate atomization models for LES.
Electrohydrodynamic Atomization
![]() |
Over the past decade, there has been considerable interest in controlling the emissions from small engines in the size range of 200 cm3 or smaller. Fuel injection schemes may reduce the incidence of pollutant emissions. However, the cost of implementation is a barrier to large scale adoption. The cost of fuel injection is driven by both the need to pump the fuel to the injection pressure and to add parts for the injector head. One approach to small-scale fuel injection is to capitalize upon the benefits of electrohydrodynamics (EHD) and enhance fuel atomization. There are many possible benefits to EHD aided atomization for combustion, such as smaller droplets, wider spray cone, and the ability to control or "tune" the spray for improved performance.
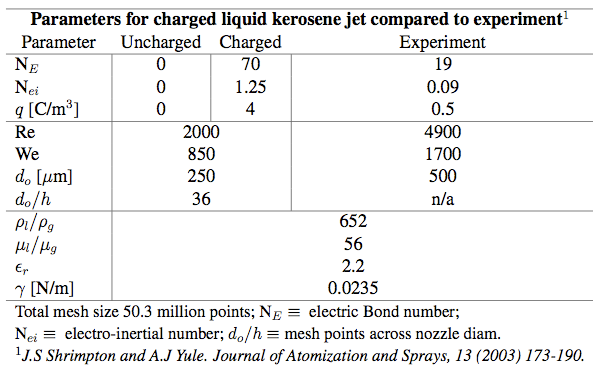
Electrohydrodynamic flows and sprays have drawn increasing interest in recent years, yet key questions regarding the complex interactions among electrostatic charge, electric fields, and the dynamics of atomizing liquids remain unanswered. Direct numerical simulations (DNS) of realistic liquid break-up are challenging due to the computational expense involved. High-fidelity numerical simulations should be able to provide some assistance in answering questions about the fundamentals and dynamics of EHD.
Recently, the NGA code has been adapted with an EHD module to explore EHD atomization. A ghost fluid method (GFM) approach is employed to solve for the electric potential Poisson equation in a sharp, accurate and robust manner. EHD boundary and jump conditions are implemented using a similar methodology, and subsequently used to solve the pressure Poisson equation. Electric charge is modeled in the bulk, with an initial assumption of no surface charge. This assumption corresponds to a regime of high electric Reynolds number, Ree=τe/τf, where τe is the charge relaxation time scale and τf is the fluid advection time scale. We will use this new module to perform large-scale, high fidelity DNS simulations of EHD atomization.